Complex materials and industrial mathematics
Our research
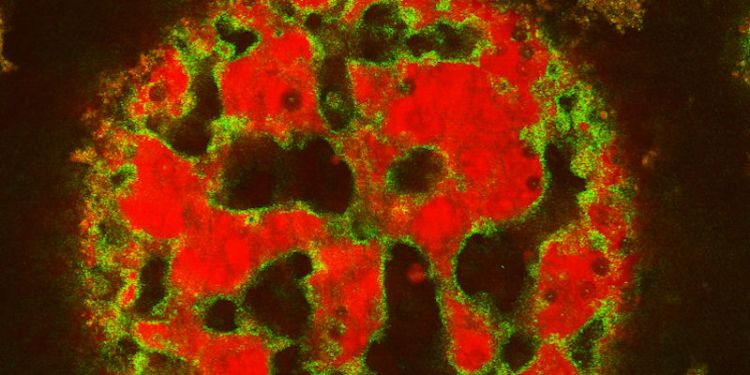
Our research covers the following areas:
- Polymer dynamics and rheology
- Inverse problems
- Non-Newtonian fluids
- Thin-film industrial (coating) and ophthalmic (tear-film) flows
- Novel continuum algorithms for mesoscale biosimulation
- Reaction chemistry and branched polymer architecture
- Polymer dynamics and neutron scattering
- Suspension mechanics
- Crystalline deposits
- Shale gas
- Non-equilibrium statistical mechanics of soft matter
- Dynamics of polydisperse substances
- Optimisation of genetic algorithms for chemical process design
Polymer dynamics and rheology
Polymers are long molecules made from joining together lots of small molecules (or monomers). Sometimes polymer molecules are linear, but very often - notably in the case of Low Density Polyethylene (LDPE) used to make plastic bottles - they include many branches. During the manufacture of polymeric (or plastic) materials and commodities, liquids containing polymers are subjected to flow. The way these liquids react is determined by the shapes, or configurations that the molecules adopt. Polymer molecules behave like springs, and become stretched by the flow, giving rise to the strongly elastic behaviour of polymeric fluids. The study of the dynamics of polymer molecules is very important for the understanding of flow of polymeric fluids. If polymer molecules overlap sufficiently, then they get tangled up (like spaghetti) so that they are constrained in their movement. The "tube model" for entangled polymers provides a conceptual framework for understanding the constrained motion, and for making mathematical predictions about the polymers' response to flow. Branchpoints in the polymer molecules provide additional obstacles to the motion of entangled polymers, so that the distribution of branchpoints in polymer molecules can be a critical factor in determining flow properties.
Inverse problems
Whilst direct formulations consist of determining the effect of a given cause, in inverse formulations the situation is completely, or partially reversed. The interest is into the research of inverse problems for partial differential equations governing phenomena in fluid flow, elasticity, acoustics, heat transfer, mechanics of aerosols, etc. Typical practical industrial applications relate to flows in porous media, heat conduction in materials, thermal barrier coatings, heat exchangers, corrosion, etc. The objectives are to investigate the existence, uniqueness and stability of the solution to the problem that mathematically models a physical phenomenon under investigation, and to develop new convergent, stable and robust algorithms for obtaining the desired solution. The analyses concern inverse boundary value problems, inverse initial value problems, parameter identification, inverse geometry and source determination problems. The determination of heat transfer coefficients (in heat conductors and polymers) using inverse methods is supported by a prestigious EU-FP7 Marie Curie International Incoming Fellowship. We are represented on the Academic Advisory Board of the UK Knowledge Transfer Network for Industrial Mathematics and the Smith Institute and for the past 5 years we have organised Industrial Mathematics Inverse Problems Sandpits at which people from industry presented their problems. This resulted in numerous industrial contacts being made, consultancy work being performed, and several EPSRC Industrial Mathematics Cases being awarded.
Non-Newtonian fluids
Elastic stresses in polymeric and other complex fluids can give rise to strange flow behaviour not seen in Newtonian fluids. This can, for example, produce undesirable instabilities in industrial processes. The aim of our research is to predict how these fluids will flow in various flow geometries and to determine the conditions for the flow to become unstable. Our current research includes investigations of how bubbles grow in polyurethane foams; how filaments of polymer stretch and break-up; and flow instabilities in extrusion. We also study instabilities in bulk suspensions and draining suspensions.
Thin-film industrial (coating) and ophthalmic (tear-film) flows
Many industrial processes and natural phenomena involve the flow of thin liquid films, perhaps the most widespread industrial application being the deposition on solid surfaces of a thin protective and/or decorative coating. After initial application, the film is usually uneven and hence evolves under competing influences such as gravity, surface tension and inertia. It is frequently required that the final (steady) state of the coating should be a film of uniform thickness, and so it is of practical value to be able to formulate and solve the nonlinear spatio-temporal evolution equations that govern the coating thickness in order to understand and predict the transient dynamics of the film. Our research in this area has led to the development of fully automated computer-algebra procedures for deriving such equations, in which our distinctive automation permits a more complete mathematical modelling of the underlying physics than has hitherto been possible. The equations have moreover been solved to a high (prescribed) degree of accuracy using similarly automated solution methods based on mutiple-timescale asymptotics. As a result, a detailed understanding of how flow evolution explicitly depends upon various physical parameters has been gained. For example, the formation and dissipation of thin-film shocks (in collaboration with Professor John Hinch FRS and Dr Paul Metcalfe, DAMTP) has been explicitly parameterized for roll-coating geometries, in which new limit-cycle phenomena resulting from inertial effects have also been discovered (in collaboration with Dr Christian Groh, Leeds).
Closely related to the above is the study of thin tear films arising in ophthalmic flows, which are complicated by additional effects such as evaporation, a viscoelastic surfactant lipid layer and free-surface dilatation. Our recent research has involved formulating model evolution equations for the tear-film thickness and surfactant concentration that are consistent in their level of approximation, so that the above-mentioned automated procedures can be modified to account for the new geometry and physics. It is hoped that this work (in collaboration with Dr Jonathan Summers and Mr Gareth Hurst, Leeds) will ultimately contribute to a better understanding of the well-known dry-eye phenomenon.
Novel continuum algorithms for mesoscale biosimulation
Modelling the dynamics of proteins is a very computationally expensive task. A wide variety of simulation techniques exist, a popular example being Molecular Dynamics. However, such a model typically involves detailed simulation of the protein's structure at, or near to, the atomic level. It is therefore unsuitable for modelling many biological entities, in particular molecular motors composed of large protein domains (e.g. Dynein), or systems in which many very large proteins are interacting (e.g. the crowded cytoplasm).
Furthermore, there are many proteins whose atomistic structures are as yet unknown, and for which the only structural information available is the overall shape obtained through experimental techniques such as Cryo-EM (see the EMDataBase) or SAXS. Working with Sarah Harris (Physics) we have developed a meso-scale protein model which treats globular proteins as a viscoelastic continuum subject to thermal noise. The protein is discretised into a tetrahedral mesh, and the dynamics due to thermal fluctuations are resolved through Finite Element Analysis; consequently this new algorithm is called Fluctuating Finite Element Analysis (FFEA). The model is parameterised locally by the bulk, continuum properties of each region of the protein: namely the shear viscosity, bulk viscosity, shear elasticity, bulk elasticity and density. The model will also accommodate intermolecular interactions such as van der Waals interactions and electrostatics, which enables the dynamics of multiple coarse-grained proteins to be modelled. Our ultimate aim is to develop FFEA into a user-friendly software tool for protein simulation that we will make available to the biophysics community.
Reaction chemistry and branched polymer architecture
There are different chemical routes used to produce branched polymers in an industrial setting. The particular reaction chemistry, and the reactor type and conditions, have a large effect on the number and distribution of branches throughout the polymer molecules. Current research is examining this relationship between reaction chemistry and branched polymer architecture with a view towards the reaction design for polymer melts with tailored flow properties.
Polymer dynamics and neutron scattering
It is important to understand the shapes, or conformations that polymers take under flow conditions. Although polymer rheology (the stress response of the fluid) is one way of probing this, it is important to have other independent tools to check that the theory is right. A more direct measure of polymer shape is obtained via neutron scattering from polymers that are parially labelled with deuterium. We aim to predict neutron scattering patterns from deformed polymer melts.
Suspension mechanics
Another important class of non-Newtonian fluids is suspensions of solid particles, such as spheres and fibres, or droplets or bubbles in Newtonian or non-Newtonian fluids. For example, short glass and carbon fibres are often added to injection moulded plastics to reduce cost and to improve the mechanical or thermal properties of the finished product. Small spheres are often suspended in a fluid in order to transport, for example, pharmaceutical powders around processing plants; and many food-stuffs and skin-care products are formed from oil-in-water emulsions. While the motion of single particles in Newtonian fluids is well understood, suspensions of large numbers of particles that interact through the fluid remain challenging, both analytically and numerically.
Our current research includes investigations of how rough particle surfaces can affect the flow of a suspension of solid spheres; how collisions between fibres affect the flow properties of concentrated fibre suspensions; propagation of sound waves through colloidal suspensions; and how droplets deform in a polymeric fluid flow. When a suspension becomes very concentrated, its viscosity rises until, at the so-called “glass transition”, is refuses to flow, and will support shear stresses indefinitely. At higher concentration still, the suspension “jams”, and cannot be further concentrated. The approach to the glass transition and to the jamming transition have universal features, independent of the exact type of suspension. Understanding how these two transitions are related, and can be modified by applied forces, has implications for the processing of food, cosmetics slurries and aggregates. Computer simulations become difficult in the glassy and jamming regimes, due to the long time-scales, large numbers of particles and singular forces involved. We study the transitions by a combination of careful simulation, theory, and comparison with the experiments of Johan Mattsson (Physics).
Crystalline deposits
Crystallisation is a key underlying physical process in a wide range of industries, e.g. food, pharmaceutical, nuclear. The demand for models that can accurately describe a crystallisation process is very high because it can offer a range of industrial benefits, such as developing an understanding of how particular parameters or process equipment can influence crystal growth, hence allowing manufacturers to optimise yield and quality of produced crystals. In collaboration with Drs Duncan Borman and Robert Hammond from Engineering, Dr Dominic Rhodes from the National Nuclear Laboratory and the EPSRC CASE Award PhD student Michael Dawson, we are currently adapting a technique used for the purpose of modelling geological stalagmite formations in order to predict the morphology of crystalline droplets of liquors which can lead to the build-up of potential harmful substances.
Shale gas
Gas flow in shale is a rather poorly understood and potentially complex geophysical phenomenon. It is currently being investigated using a variety of techniques including transient experiments conducted on full core and crushed shale using a range of gases. Flow mechanisms include continuum flow, slippage, transitional flow and Knudsen diffusion. These processes, as well as gas sorption, need to be taken into account when interpreting experimental data and extrapolating the results to the subsurface. In collaboration with Dr Piroska Lorenczi and Professor Quentin Fisher from the Centre for Integrated Petroleum Engineering we are currently modelling the transient gas in shale through a pressure-pulse decay test using a nonlinear diffusion model incorporating the Knudsen number, in which the permeability and fluid density depend on the pressure. Apart from validating the mathematical model for the direct problem against real measurement data, the inverse problem of determining several physical parameters such as the intrinsic permeability, tortuosity and its compressibility coefficient from transient measurements of the upstream pressure is analysed.
Glomerular filtration rate
Chronic kidney disease (CKD) is a condition characterised by a gradual loss of kidney function over time. It is a big health issue in the US because it affects more than 10% of its population. At present, glomerular filtration rate (GFR) is clinically the most used measure of renal function in CDK. It has been recently validated that based on rigid-body image registration, semi-automated segmentation and tracer-kinetic modelling, dynamic contrast-enhanced magnetic resonance imaging (DCE-MRI) is a suitable method for measuring the single-kidney GFR. In collaboration with Dr Steven Sourbron from Medical Physics, Dr Phil Murphy from GlaxoSmithKline, and the EPSRC CASE Award PhD student Dimitra Flouri we are trying to improve the efficacy and robustness of each of these steps by integrating them into one larger inverse problem.
Controlling crystallization using metastable phases
Crystallization takes place in a very wide variety of industrial processes, from tempering chocolate, to moulding plastics, to manufacturing silicon chips. It is also medically important, since identifying the structure of a new molecule for drug discovery, or the structure of a protein, requires the substance to be crystallized, ready for X-ray diffraction. Crystallizing an unknown substance is a hit-and-miss operation, which would be helped by better understanding and control of the processes of crystallization. Most substances can exist in “metastable phases”, i.e. states of the material that are long-lasting, but are not the ultimate thermodynamically stable state. The appearance of metastable phases often impedes crystal formation, but can also be used to control and even enhance crystallization. A recent PhD in the group, by John Williamson, has scratched the surface of this topic and demonstrated how productive its study can be.
Aggregation in foods
Almost all foods are complex mixtures of solids and fluids. The structures of the solid and semi-solid regions are responsible for the food's texture, appearance and durability. So, understanding how those structures form is key to controlling a food's appeal, mouth-feel, cost and shelf-life. In many foods, such as cheese, yoghurt and blancmange, the structures form by a ubiquitous process of particle diffusion and aggregation, resulting in a locally fractal geometry. Although some aspects of this process are well understood, it has previously been modelled only approximately, often ignoring hydrodynamic interactions between particles. This is because simulating the flow of fluid around a large number of particles requires a large amount of computing power. A recently developed algorithm, together with the High-Performance Computing facilities at Leeds, allow us to model the dynamics and to compare and contrast the resulting aggregated structures produced under different circumstances. A preliminary study is under way, in collaboration with Rammile Ettelaie (Leeds Food Science) and PhD student Craig Hall (CMIM group).
Non-equilibrium statistical mechanics of soft matter
Soft matter includes colloids, emulsions, liquid crystals, amphiphiles and polymeric fluids. It is ubiquitous, constituting virtually all foods and biological materials. Soft matter has useful properties that are exploited in industrial applications including plastics, displays, adhesives, coatings, cosmetics and countless others. In soft matter, we find an exotic menagerie of complex structures that can be understood in terms of their interesting symmetries and statistical properties. Both the microstructure and the thermodynamic properties of such materials can be investigated by statistical mechanics, the theoretical framework that connects the properties of microscopic particles to their collective behaviour en masse. But traditional statistical mechanics applies only to substances at thermodynamic equilibrium, i.e. which are quiescent, not flowing. When soft matter flows, it exhibits much more interesting behaviour than simple fluids, including flow-alignment, shear-banding, and jamming. Our research is focused on developing methods for the mathematical modelling of non-equilibrium thermodynamic systems including the application of Large Deviations Theory to non-equilibrium systems, in collaboration with members of the EPSRC Grand Challenges Network “Emergence and Systems Far from Equilibrium”. The resulting mathematical structure bears beautiful similarities to that of equilibrium statistical mechanics, and has as great potential for applications. Some of our research uses idealized mathematical models of flowing fluids, in which the molecules are replaced by simpler entities such as interacting rotors. This allows us to study some of the principles and foundations of non-equilibrium statistical mechanics in systems that are sufficiently complex to mimic some aspects of real materials, but sufficiently simple to investigate mathematically.
Dynamics of polydisperse substances
Equilibrium properties of materials become difficult to calculate if the constituent particles are not all identical, but vary in size, shape, charge etc. This is typically the case in colloidal suspensions and in polymer solutions and melts. We have developed methods for simplifying the mathematics associated with such 'polydisperse' substances, so that their phase behaviour (e.g. when they crystallize / boil / dissolve) can be understood. Current research focuses on polydisperse systems that are out of equilibrium, to model their dynamics of mixing, demixing and crystallization.
Developing microrheological techniques
Rheology is usually conducted by applying large-scale shear to the fluid under investigation, by moving parallel plates. In micro-rheology, tiny sample volumes can be studied by analysing the motion of microscopic test-beads suspended in the fluid. This motion may be either Brownian, or driven by an applied magnetic field. In an on-going collaboration with Dr M Tassieri (Glasgow University), we design new methods to extract, from the correlated motions of many probe-particles, accurate measurements of the macroscopic visco-elastic shear moduli, which are unaffected by the finite-size boundary effects that usually plague such measurements.
Optimisation of genetic algorithms for chemical process design
Through the Leeds Crystallization Centre, we collaborate with Professor Fiona Meldrum (Leeds Chemistry), to develop new methods for controlling the yield and morphology of some mineralization reactions. In novel chemical systems with a large number of control parameters, finding the optimal admixtures requires a search in a high-dimensional parameter space. We aim to accelerate that search by mimicking some of the processes of Darwinian evolution.